Authors: Cheng-Mei Yang, Tian-Huei Chu, Kuo-Wang Tsai, Shuchen Hsieh, ORCID, Mei-Lang Kung.
29 January 2022 – https://www.mdpi.com/2227-9059/10/2/320
Abstract
1. Introduction
2. Materials and Methods
2.1. Cell lines and Nanosized Zingerone
2.2. Cytotoxicity Assay
2.3. Colony Formation Assay
2.4. Invasion Assay
2.5. Wound Healing Assay
2.6. Gelatin Zymography Assay
2.7. Quantified PCR (qPCR) Analysis
2.8. Western Blot Analysis
2.9. Statistical Analysis
3. Results
3.1. Zingerone NPs Inhibited Proliferation and Suppressed Tumorigenesis in Human Oral Squamous Cell Carcinoma (OSCC) Cell Lines In Vitro
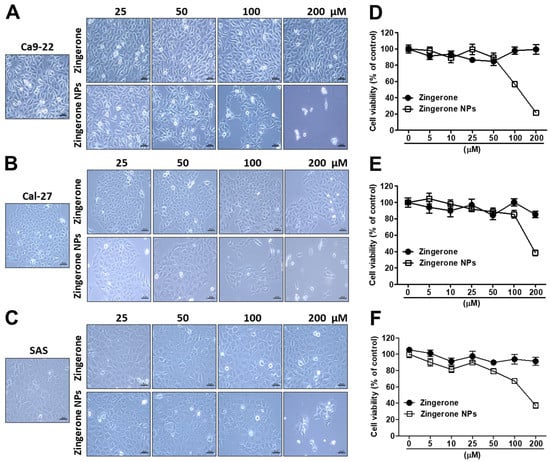
Next, cells were cultured with zingerone and/or zingerone NPs and then subjected to an in vitro colony formation assay to characterize the effect of zingerone NPs on the tumorigenicity of OSCC cells. As shown in Figure 2, compared to zingerone, zingerone NPs induced a superior inhibition of colony formation in these OSCC cell lines. In Ca9-22 cells, zingerone significantly inhibited colony formation in a dose-dependent manner. Intriguingly, even the lowest dose (10 µM) of zingerone NP treatment exhibited an obvious suppressive efficacy (Figure 2A). Similar results were also obtained using the Cal-27 (Figure 2B) and SAS cell lines (Figure 2C), although the effective dose was higher than that in Ca9-22 cells (approximately 25 µM). Moreover, the colony numbers and colony survival were further analyzed and recorded. Zingerone significantly reduced colony numbers and colony survival in these OSCC cell lines in a dose-dependent manner. Undoubtedly, zingerone NPs elicited more substantial decreases in colony numbers and colony survival. Even at the lowest dose (10 µM), zingerone NPs significantly decreased colony numbers by greater than 60% and colony viability by 70%, and the higher dose (over 25 µM) treatment induced approximately a 100% inhibition of colony survival in Ca9-22 cells (Figure 2D). A similar outcome was also observed in Cal-27 cells (Figure 2E) and SAS cell lines (Figure 2F), indicating that a lower dose of treatment (25 µM) dramatically decreased colony formation and colony viability by greater than 80%. The complete inhibition of colony activity was observed in cells treated with doses greater than 50 µM. Therefore, zingerone NPs inhibited the proliferation and tumorigenicity of OSCC cell lines.
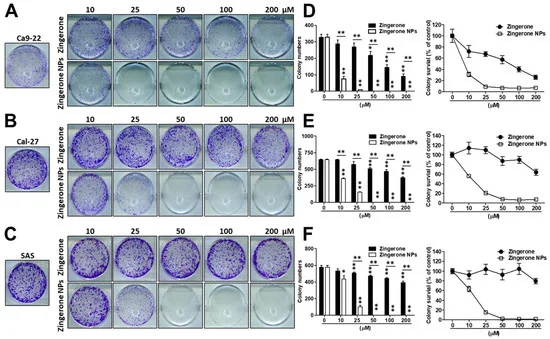
3.2. Zingerone NPs Attenuated the Migration and Invasion of Human OSCC Cell Lines
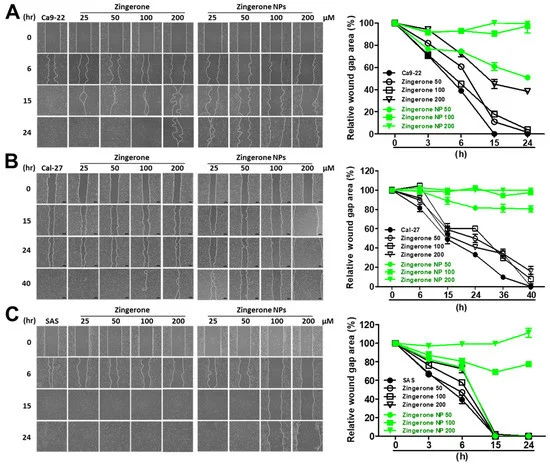
Next, the effect of zingerone NPs on cell invasion was also examined. Cells were mixed well with zingerone and/or zingerone NPs and then plated in a Boyden chamber equipped with a Matrigel-coated membrane. After an adequate incubation time, cellular invasion was analyzed and recorded. Compared to zingerone, zingerone NPs dramatically inhibited the invasion of these OSCC cell lines in a dose-dependent manner (Figure 4A–C). In Ca9-22 cells, zingerone NPs inhibited invasion by 20–60% in a dose-dependent manner compared to the zingerone group, which did not reach over 20% inhibition. Even the lowest dose (25 µM) of zingerone NP treatment induced 20% inhibition, which was only achieved with the highest dose (200 µM) of zingerone treatment (Figure 4D). In Cal-27 cells, zingerone NPs noticeably decreased invasion by 50–95% in a dose-dependent manner compared to zingerone, which did not substantially affect cell invasion (Figure 4E). In SAS cells, zingerone NPs inhibited invasion to a similar extent as that in Ca9-22 cells (Figure 4F). These results suggested that zingerone NPs exhibited a superior ability to inhibit the invasion of human OSCC cells.
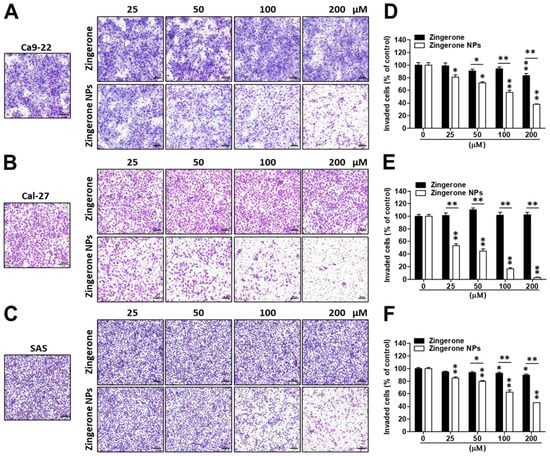
3.3. Downregulation of Akt Signaling and EMT Signaling Was Involved in Zingerone NP-Mediated Inhibition of Cell Proliferation and Metastasis
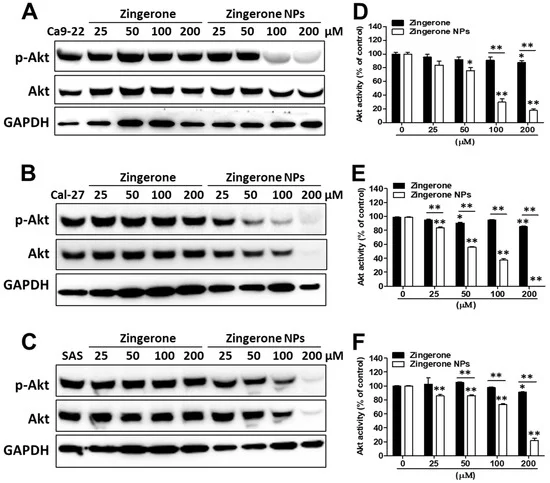
Overcoming the extracellular matrix (ECM) barrier is the first step in tumor cell invasion and distant metastasis. The enzymatic digestion of ECM by matrix metalloproteinases (MMPs) is an important component of normal physiological and pathological processes, such as embryonic development, wound healing, inflammation, and cancer [35]. In addition, due to the critical roles of MMPs in tumor cell invasion and metastasis, MMPs such as MMP2 and MMP9 have been identified as prognostic factors for many types of cancer, such as head and neck squamous cell carcinomas (HNSCCs) and oral cancers. [36,37] Therefore, we further analyzed the effects of zingerone NPs on MMP activity. Using a zymography assay, we found that zingerone NPs significantly attenuated MMP2 and MMP9 activity in these three OSCC cell lines compared to zingerone treatment (Figure 6A–C). Moreover, the effect of zingerone NPs on reducing the levels of lower molecular weight MMP proteins (~45–50 kDa) was also observed (Figure S3). Additionally, compared to MMP9, zingerone NPs seemed to be more effective at decreasing MMP-2 activity in a dose-dependent manner. Accordingly, we further analyzed the expression level of the MMP2 mRNA using quantitative PCR analysis. In Ca9-22 cells, zingerone significantly increased MMP2 mRNA levels. Conversely, zingerone NPs dramatically suppressed MMP2 mRNA expression in a dose-dependent manner, and the effective dose for MMP2 mRNA inhibition was observed with the 50 µM treatment (Figure 6D). A similar result was also observed in Cal-27 and SAS cells; in particular, a greater decrease in MMP2 mRNA levels was observed after treatment with the higher dose (100 µM) of zingerone NPs (Figure 6E,F). According to these data, zingerone NPs abolished cell migration and invasion, and may simultaneously decrease the activities of various MMPs, especially MMP2 and MMP9. Indeed, several MMPs have been shown to digest gelatin, such as MMP1, MMP3, MMP10, and MMP12, whose molecular weights range from approximately 54 to 57/44 to 45 kDa in latent/active forms, respectively [38]. Moreover, these MMPs also play roles in various types of cancer and metastasis [39].
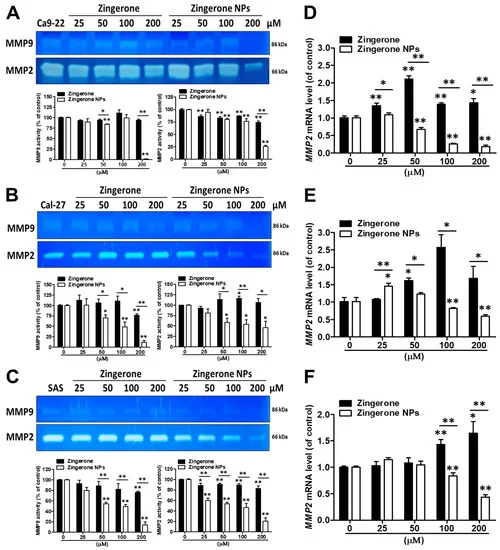
On the other hand, due to the superior efficacy of zingerone NPs in suppressing cellular invasion, we next investigated the effects of zingerone NPs on the levels of EMT-related markers, such as the epithelial markers ZO-1 and E-cadherin, and the mesenchymal markers N-cadherin and vimentin. In Ca9-22 cells, zingerone NPs significantly reduced the levels of the mesenchymal markers of N-cadherin and vimentin, rather than the epithelial marker of ZO-1, in a dose-dependent manner compared with zingerone. Moreover, neither zingerone nor zingerone NPs induced an obvious change in the epithelial marker of E-cadherin protein levels (Figure 7A,B). Interestingly, in Cal-27 cells, zingerone achieved maximal reductions of 30% and 45% in the levels of epithelial markers (ZO-1 and E-cadherin) and mesenchymal markers (vimentin, but not N-cadherin), respectively. In contrast to zingerone, zingerone NP treatment induced substantial decreases in the levels of both epithelial markers and mesenchymal markers in a dose-dependent manner (the maximal inhibition achieved was >90%) (Figure 7C,D). Moreover, in SAS cells, zingerone treatment induced a slight increase in the levels of mesenchymal markers (N-cadherin and vimentin) compared with epithelial markers (E-cadherin, but not ZO-1). Conversely, zingerone NPs significantly reduced the levels of both epithelial markers and mesenchymal markers, and the effective dose was at least 100 µM, which achieved a decrease of approximately 60% (Figure 7E,F). These results revealed that zingerone NPs substantially altered the levels of EMT-related markers, and especially interfered with mesenchymal markers compared to epithelial markers among these OSCC cell lines.
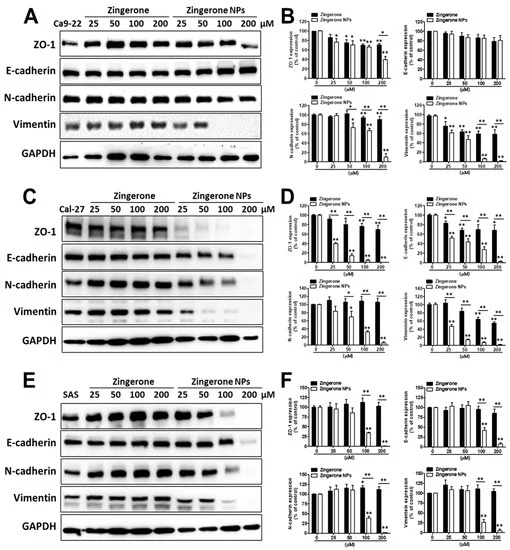
4. Discussion
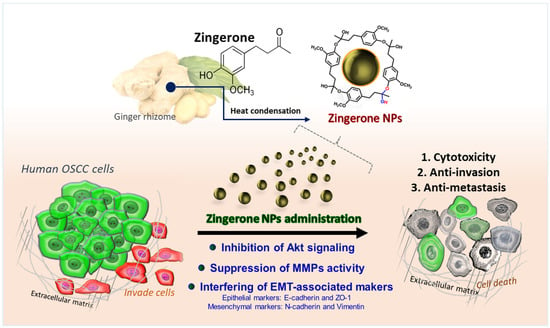